When Bivalves Attack or Bivalves Vs Birds the Battle Continues
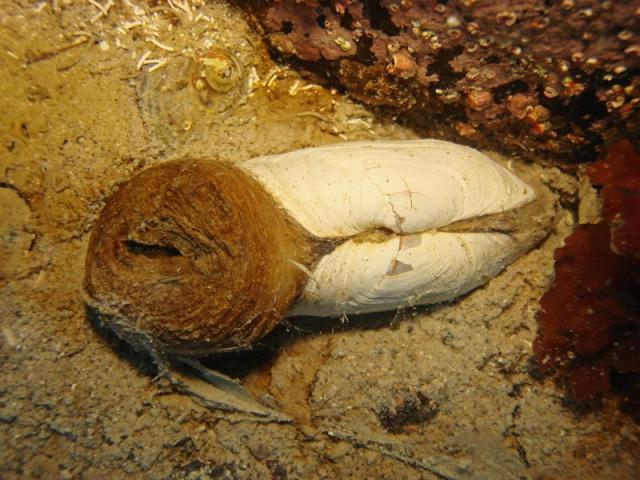
Like all organisms, bivalves have a limited budget governing all aspects of their metabolism. If they put more energy into feeding (filtering the water), they can bring in a bit more food and therefore fuel more growth, but sucking in water takes energy as well, particularly if there isn't enough food to be filtered out. Bivalves also periodically have to grow gonadal material and eggs for reproduction, expand their body tissue (somatic growth) and of course, grow their shells (made of of a mineral called carbonate). All of these expenditures are items in a budget determined by the amount of energy the bivalve can bring in, as well as how efficiently they can digest and metabolize that energy.
If a bivalve is placed under stress, their scope for growth (the max amount of size increase per unit time) will be decreased. Because they're cold-blooded, bivalves are limited by the temperature of their environment. If temperatures are low, they simply can't sustain the chemical reactions required for life at the same rate that endotherms like us can. They also may have to shut their shells and stop feeding if they're exposed by the tide, or are tossed around by a violent storm, or attacked by predators or toxins from the algae that they feed on.
When their budget is lower, they have to make painful cuts, much like a company lays off employees if their revenues are lower. The question is which biological processes get cut, and when? My first chapter (submitted and in review) has settled temperature being the primary control on seasonal shell growth. Bivalves at high latitudes undergo annual winter shutdowns in growth, which create the growth bands I use to figure out their age, growth rate, etc. We'd be a lot closer to accurately predicting when bivalves suffer from "growth shutdowns" if we had hard numbers on how much energy they actually invest in their shells. A new study from a team led by Sue-Ann Watson of James Cook University attempts to do just that.
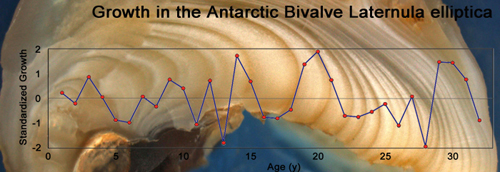
Collecting a database of widths for the annual growth rings of bivalve and gastropod (snail) species from many latitudes, Watson and her team were able to get a global view of how fast different molluscs grow from the equator to the poles. Because the unit cost of creating carbonate is determined by well-understood chemistry, they were able to create an equation which would determine the exact number of Joules of energy used for every bivalve to grow their shells.
They still needed a total energy budget for each species, in order to the percent of the energy budget that each bivalve was investing in their shells. They drew on a previous paper which had calculated the standard metabolic rates for each species by carefully measuring their oxygen consumption. We could do the same for you if you sat in a sealed box for an extended period of time while we measured the exact amount of oxygen going in and CO2 going out. Dividing the amount of energy needed to grow the shell by the total amount of energy used in the organism's metabolism would give us a percent of total energy that the bivalve dedicates to adding growth layers to its shell.
That number is…not very large. None of the bivalves or gastropods they looked at put more than 10% of their energy into shell growth, and bivalves were the lowest, with less than 4% of their energy going into their shell. Low-latitude (more equatorward) bivalves have the easiest time, putting less than 1% of their energy into growth but getting way more payoff for that small expenditure. High-latitude polar bivalves have to work harder, because the lower temperatures they experience mean the reactions needed to create their shells are more expensive. In addition, most of that energy is going into the protein-based "scaffolding" that is used to make the shell, rather than the crystals of carbonate themselves. Organisms right now don't have to put a whole lot of effort into making their protective shells, which could explain why so many organisms use shells for protection. That is good, because if shells were already breaking the bank when it came to the bivalves' growth budget, they wouldn't have a lot of room to invest more energy in the face of climate change. Unfortunately, as the authors note, these budgets may need to change in the face of climate change, particularly for bivalves at the poles. As the oceans grow more acidic due to human CO2 emissions, growing their shells will start to take up more of their energy, which is currently not a major part of their budget.
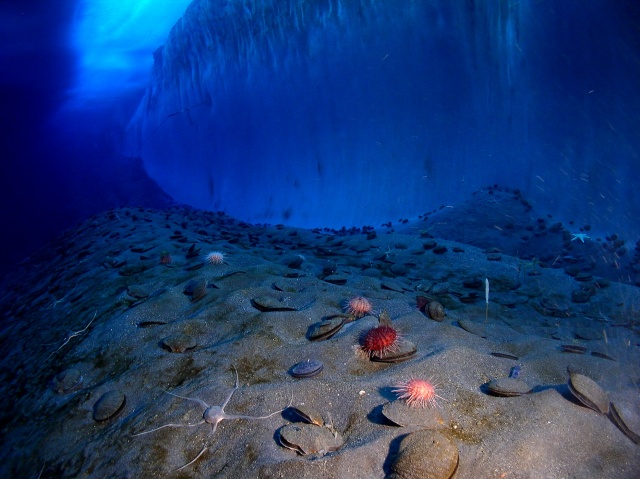
Right now, the cold waters of the poles are refuges for organisms that don't deal well with shell crushing predators. As polar regions warm, such predators will begin to colonize these unfamiliar waters. Polar bivalves may encounter the double whammy of needing to spend more energy to make the same amount of shell, but also find that it is no longer enough to protect them from predators that easily crack open their protective coverings.
I found this study to be an elegant and thoughtful attempt to fill in a gap in our current understanding of how organisms grow and how energy budgets are influenced by environmental variables like temperature. I instantly downloaded the paper because it answered a question that has long been on my mind. Maybe can sneak its way into my manuscript during the review process!
Source: https://dantheclamman.blog/2017/10/03/hard-shells-arent-actually-that-hard-to-make-yet/
0 Response to "When Bivalves Attack or Bivalves Vs Birds the Battle Continues"
Post a Comment